Introduction
Geological setting of the study area
Research methods
Results
Site 1
Site 2
Site 3
Discussion
Conclusion
Introduction
Magma is transported from its source to Earth’s surface via vertical or sub-vertical fractures that can feed volcanoes. However, not all magmas are erupted, with the vast majority of them remaining trapped within the crust (Crisp, 1984; Shaw, 1985). Dikes, a type of magmatic intrusion, can be used to study paleo-stress in Earth’s crust, as it is generally accepted that dikes intrude in the direction normal to the minimum principal stress, σ3 (Anderson, 1951). However, dikes also commonly intrude along pre-existing weak structures such as faults and joints, in which case it is difficult to decipher the direction of minimum principal stress. In such cases, the method of matching piercing points located on the two sides of a dike is useful for inferring the paleo-stress that applied during intrusion (e.g. Yang et al., 2008). It is clear, therefore, that the tectonicstress conditions as well as the directions of pre-existing fractures influence the geometry and propagation direction of dikes (Odé, 1957; Muller and Pollard, 1977; Pollard, 1987; Rubin, 1995; Dahm, 2000; Mériaux and Lister, 2002; Geshi, 2005; Gudmundsson and Phillip, 2006; Acocella and Neri, 2009; Siler and Karson, 2009; Schofield et al., 2010). In addition, some studies have shown that the direction of dike intrusion also depends on dike buoyancy (e.g., Lister and Kerr, 1991; Dahm, 2000; Mériaux and Lister, 2002), which in turn depends on the density difference between rock and magma and the vertical gradients of the normal external deviatoric stress (Takada, 1989).
Pre-existing fractures, including faults, commonly act as conduits for the passage of magma. Dikes can intrude along pre-existing structures (e.g., fractures, joints, and faults) involving dilation (Delaney et al., 1986; Tokarski, 1990; Gudmundsson, 2011) and can be deflected along bedding to form sills (Gudmundsson, 2006). If a dike intrudes along pre-existing fractures, the opening direction based on piercing points can be used to infer the direction of minimum principal stress during intrusion (Anderson, 1951; Odé, 1957; Zoback and Zoback, 1980; Best, 1988; Ernst et al., 1995).
Kinematic analyses of faults can also provide useful information on paleo-stress (e.g., Kim et al., 2003; Wilson et al., 2003; Crider and Peacock, 2004; Blenkinsop, 2008). The paleo-stress condition can be inferred on the basis of the orientations of faults or associated fractures and their slip senses (Wallace, 1951; Bott, 1959; Gushchenko, 1975; Angelier and Mechler, 1977; Parfenov, 1981; Angelier, 1984; Lisle, 1987; Nemcok and Lisle, 1995; Rebetsky, 1997; Yamaji, 2000; Célérier et al., 2012; Fossen, 2016). In previous studies, paleo-stress has been analyzed from conjugate faults (Anderson, 1951; Gzovsky, 1954; Gzovsky et al., 1972), shear zones (Hansen, 1971; Cowan and Brandon, 1994) and fractures and veins (e.g., Nickelsen and Hough, 1967; Nikolaev, 1977; Engelder and Geiser, 1980). Structural events can be reconstructed from stress fields inferred from the orientations of faults and dikes, and knowledge of the stress field can be used to estimate fault reactivation (Morris et al., 1996) and to understand the tectonic history of the area of interest (e.g., Jamison, 1992; Van der Pluijm, 1997; Cardozo et al., 2016).
The relationships between dike geometry, distribution, structural deformation, emplacement mechanism, and timing of emplacement can be used to identify and sequence deformation events (Halls, 1982; Ernst et al., 1995; Li, 2000; Marinoni, 2001; Bleeker and Ernst, 2006; Hou et al., 2006, 2010; Goldberg, 2010; Ernst, 2014) and hence contribute to deducing the tectonic history of the area of interest. The study reported here aimed to decipher the deformation history of rocks in Geoje Island, Korea, using information measured from dikes and faults within the study area. This paper presents field data collected during the study, interprets the data to identify deformation events, and relates the events to regional fault system reactivation under changing stress regimes and to the tectonic history of the Korean Peninsula.
Geological setting of the study area
The Korean Peninsula is located in the eastern margin of the Eurasian continent. During the Cretaceous, the eastern Eurasia was subjected to a sinistral transcurrent tectonic regime related to the subduction of the proto-Pacific plate beneath the Eurasia. This sinistral regime was characterized by periods of fault reactivation and led to the formation of back-arc basins and strike-slip faults oriented parallel to the Eurasian plate margin (Chun and Chough, 1992; Kinoshita, 1995; Kim et al., 1997).
The study area, Geoje Island, is located in the Gyeongsang Basin, which is the largest continental basin in Korea and is located in the southern part of the northeast-trending Yangsan fault system (Fig. 1). The basin fill comprises the Gyeongsang Supergroup and is composed of nonmarine strata with interlayered volcanic products and plutonic rocks (Choi, 1986; Chang et al., 1997). The Gyeongsang Supergroup is subdivided into three groups, namely, the Sindong, Hayang, and Yucheon groups. The Sindong and Hayang groups are dominated by mudstones, sandstones, and conglomerates that were deposited in fluvial, alluvial, and lacustrine environments, whereas the Yucheon group is composed mostly of volcanic rocks with felsic to intermediate compositions (Chang, 1975, 1977; Chang et al., 1997).
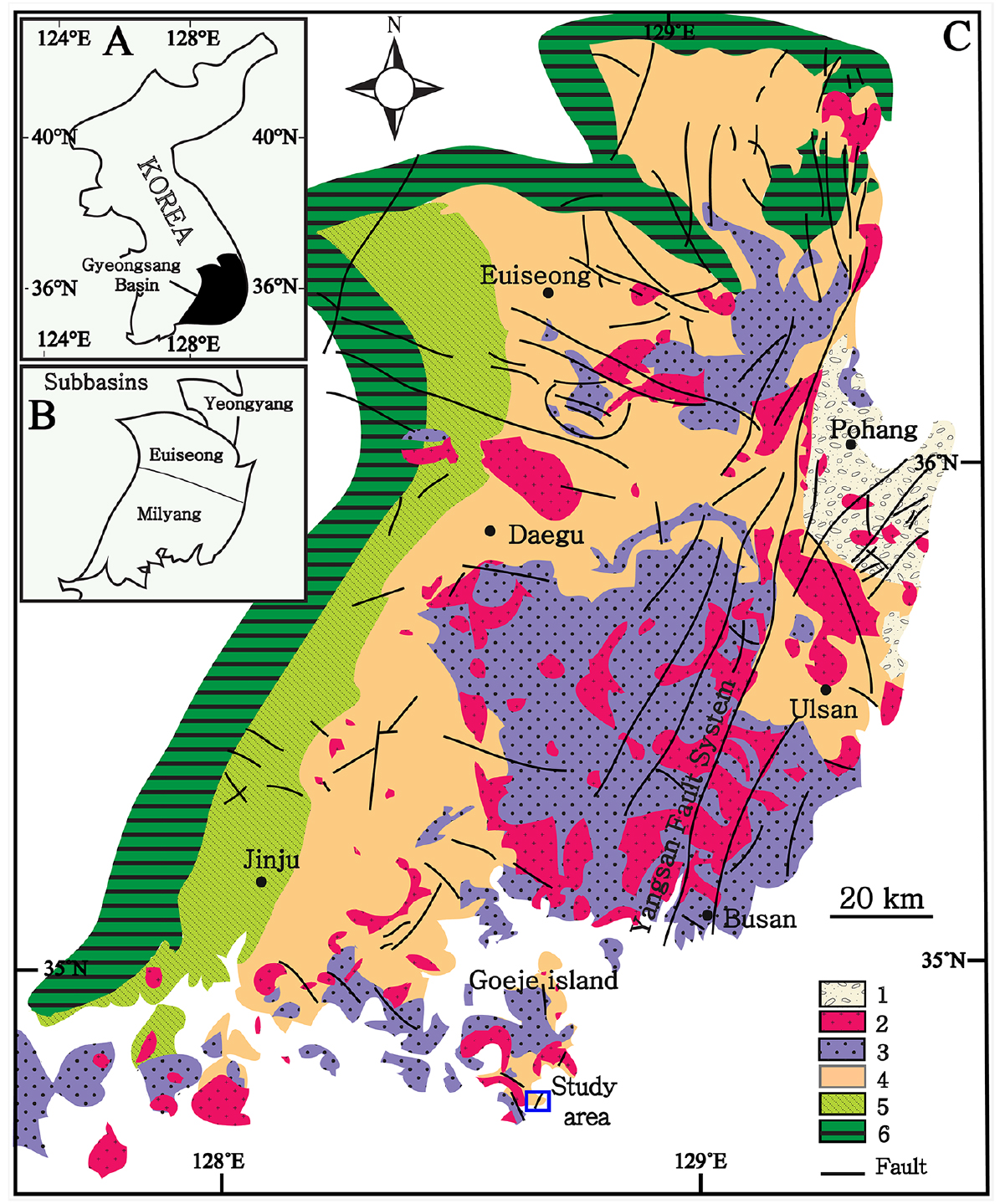
Fig. 1.
Location and geological map of the Gyeongsang Basin (modified from Lee and Lee, 2001). A. Location of Gyeongsang Basin on the Korean Peninsula. B. Subbasins of the Gyeongsang Basin separated by WNW-ESE-trending faults. C. Geological map of the Gyeongsang Basin.1. Tertiary sedimentary/volcanic rocks. 2. Late Cretaceous-Paleogene plutonic rocks. 3. Late Cretaceous Yucheon Group. 4. Early Cretaceous Hayang Group. 5. Early Cretaceous Sindong Group. 6. Pre-Cretaceous metamorphic/plutonic rocks.
The Gyeongsang Basin is tectonically divided into the Yeongyang, Uiseong, and Miryang subbasins from north to south (Chang, 1977). These subbasins are cut by several systematic fault sets, which have been used to constrain the origin and evolution of the Gyeongsang Basin. In the Uiseong block, the Gaum fault system, which comprises WNW-ESE-trending sinistral strike-slip faults, predominates, whereas the Miryang Block is transected by NNE-SSW-trending dextral strike-slip faults of the Yangsan fault system. The two fault systems do not intersect. Lee and Hwang (1997) suggested that these fault systems might have been produced by diachronous geological events and concluded that the Yangsan fault system was generated after the Gaum fault system. In contrast, Hwang et al. (2007a) suggested that these fault systems could be coeval on the basis of a model of conjugate fault evolution by block rotation in opposite directions (Ron et al., 1984; Nur et al., 1986).
The Seongpo-ri Formation in the study area is correlated with the upper Albian to lower Cenomanian rocks of the upper Haman Formation of the Hayang Group (Lee and Lim, 2008). The study area is located at the southern part of the reported Yangsan fault system (Fig. 1), where hornfelsic rocks predominate owing to the intrusion of stock-shaped granitic bodies and intermediate-composition dikes.
Research methods
For this study, three sites were selected for field data collection (Fig. 2). The aim of the structural analysis was to measure and understand the geometry and kinematics of brittle or hydroplastic structures, such as faults, extensional fractures, joints, veins, and dikes. Dike orientations were measured together with the structural attitudes of other structural features. Structural data were grouped into several sets based on their orientations, geometries, kinematics, observed structural signatures, and relationships. Sets of structural data were interpreted as having formed by different deformation events on the basis of their kinematic consistency. Rose diagrams and contour diagrams of equal-area, lower-hemisphere stereographic projections were used to analyze the structural data.
Results
In this study, we identified 34 magmatic dikes, which can be classified into 5 sets based on their orientations and crosscutting relationships: NNE-SSW-, NE-SW-, NW-SE-, NNW-SSE-, and E-W-trending dikes. Strike-slip faults are dominant in the study area, with 35 strike-slip faults being identified within intrusive dikes and host sedimentary rocks. These faults can be grouped into NW-SE-striking sinistral and dextral, NNW-SSE-striking sinistral, E-W-striking dextral, and NE-SW-striking sinistral and dextral faults. Data for faulting events and dike intrusions were combined to accurately interpret the deformation events. It is possible that the NW-SE-trending sinistral and E-W-trending dextral strike-slip faults are conjugate faults. NE-SW-oriented faults encompass both sinistral and dextral strike-slip faults with σ1 oriented NNE-SSW and E-W, respectively, but there is no direct evidence for the order of the two events.
Site 1
At this site, dike 2-1 oriented NE is intruded within Cretaceous sedimentary rocks with constant thickness of the dike, and no piercing points are observed. This dike experienced several deformation events as it has been deformed by faults and crosscutting dikes (Fig. 3). Because this dike shows no piercing points, the opening direction is assumed to be perpendicular to the strike of the dike. Therefore, we infer that the intrusion of dike 2-1 was not controlled by pre-existing structures. Dike 2-1 has been displaced by both sinistral and dextral strike-slip faults, but the chronology of these faults is not clear due to limited evidence. Dike 2-1 has been intruded by dikes 4-1 and 3-2-1 (Fig. 3A). There are two possibilities for the intrusion of dikes 4-1 and 3-2-1: 1) They may have been intruded within a NNW-SSE-striking sinistral strike-slip fault and NW-SE-striking dextral strike-slip fault, respectively; or 2) they created their own paths of intrusion and the faults formed during a later event. The lack of slip surfaces and other kinematic indicators overprinting the dikes suggests that the dikes intruded within pre-existing faults. In addition, no piercing points were observed on either of these dikes, which is attributed to the fact that the dikes have been eroded, with only partial dikes remaining in the outcrop. Therefore, we suggest that the intrusion of dikes 3-2-1 and 4-1 was controlled by pre-existing sinistral and dextral strike-slip faults, respectively.
Two sets of conjugate faults, NW-SE-striking sinistral and E-W-striking dextral strike-slip faults, are developed in dike 2-1, offsetting one another with an intersection angle of 52° (Fig. 3B). These conjugate faults represent another deformation event after the intrusion of dike 2-1. Dike 3-1-1 shows no evidence for control of its intrusion orientation/geometry by pre-existing structures, but it is cut by a sinistral strike-slip fault (Fig. 3C), as shown by the pull-apart structure around it (Fig. 3D). The intersection point of the dike and the fault is highly fractured, suggesting that the fault occurred after intrusion of the dike (Fig. 3D).
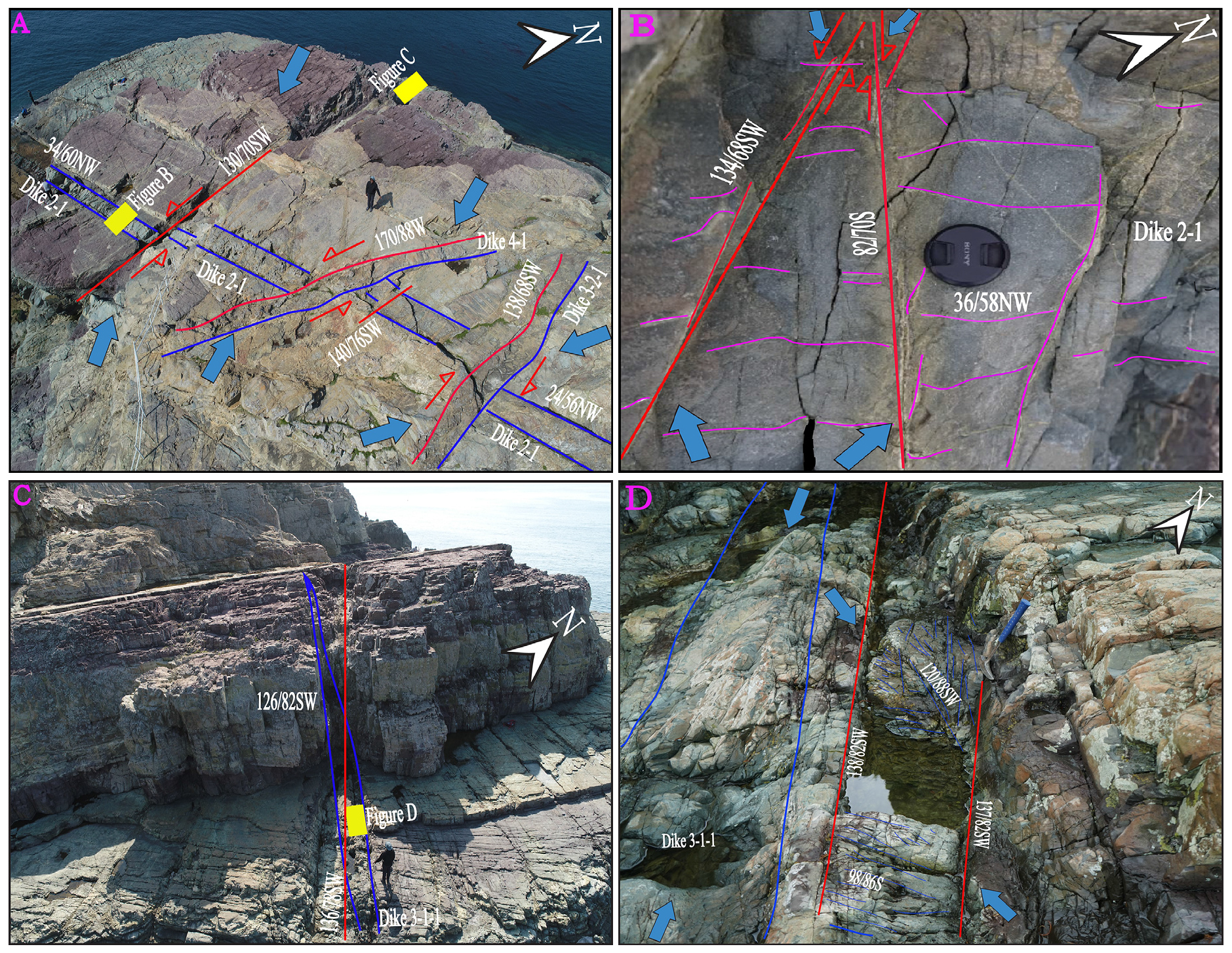
Fig. 3.
Field photographs taken at site 1. A. Dike 2-1 is intruded by dikes 4-1 and 3-2-1 through sinistral and dextral strike-slip faults, respectively. B. Two conjugate faults cutting dike 2-1. C. NW-SE-oriented dike 3-1-1 is cut by a NW-SE-striking sinistral strike-slip fault. D. NW-SE-striking sinistral strike-slip fault overprinting dike 3-1-1, with a pull apart structure formed by the linkage of two fault segments. Blue arrows indicate the direction of maximum principal compressive stress. Red arrows indicate the sense of movement. Thick, solid blue lines represent dike contact planes; thin, solid blue lines indicate tip damages; red lines depict faults; and purple lines represent veins.
Site 2
Similar to site 1, this site presents deformation events, as evidenced by vein displacement, pull-apart structures, and cross-cutting dikes (Fig. 4). This site is mainly composed of siltstones and shales deformed by intruded dikes and several strike-slip faulting events (Fig. 4A). Structural attitudes of dikes, faults, veins and fractures were measured and the chronological order of events was deduced using dike crosscutting relationships, and fault slip sense. NW-SE-oriented veins are displaced by both NE-SW-striking dextral (Fig. 4B) and sinistral (Fig. 4C) strike-slip faults, but there is no direct evidence for the order of the sinistral and dextral faulting. The presence of both NE-SW-striking sinistral and dextral strike-slip faults indicate that the change in stress condition resulted to the reactivation of this fault. The orientations of those two faults are similar to that of the Yangsan fault system located in the northeast of the study area, therefore, there might be a close relationship between them. Fig. 4D shows a linking zone formed by fault interaction at the segment tips, indicating a NW-SE-striking sinistral strike-slip fault.
Dike 3-2 is oriented NW-SE and has been intruded by NNW-SSE-oriented dike 4-2 (Fig. 4E). This relationship clearly shows that the two events were caused by a change in the direction of maximum principal stress from NW-SE to NNW-SSE. In Fig. 4E, the yellow lines connecting piercing points in dike 4-2 show the opening direction. Because the opening direction is perpendicular to the maximum compressive stress, the direction of maximum compressive stress for this dike is oblique to the strike of the dike. The unconnected end parts of the two segmented faults shown by the dashed blue lines near the piercing points indicate a stepping zone of fault segments (Fig. 4F). The inferred principal stress condition indicates dextral strike-slip faulting. Given the above, it is concluded that dike 4-2 intruded along dextral strike-slip faults with the maximum principal stress oriented NNW-SSE (Fig. 4F).
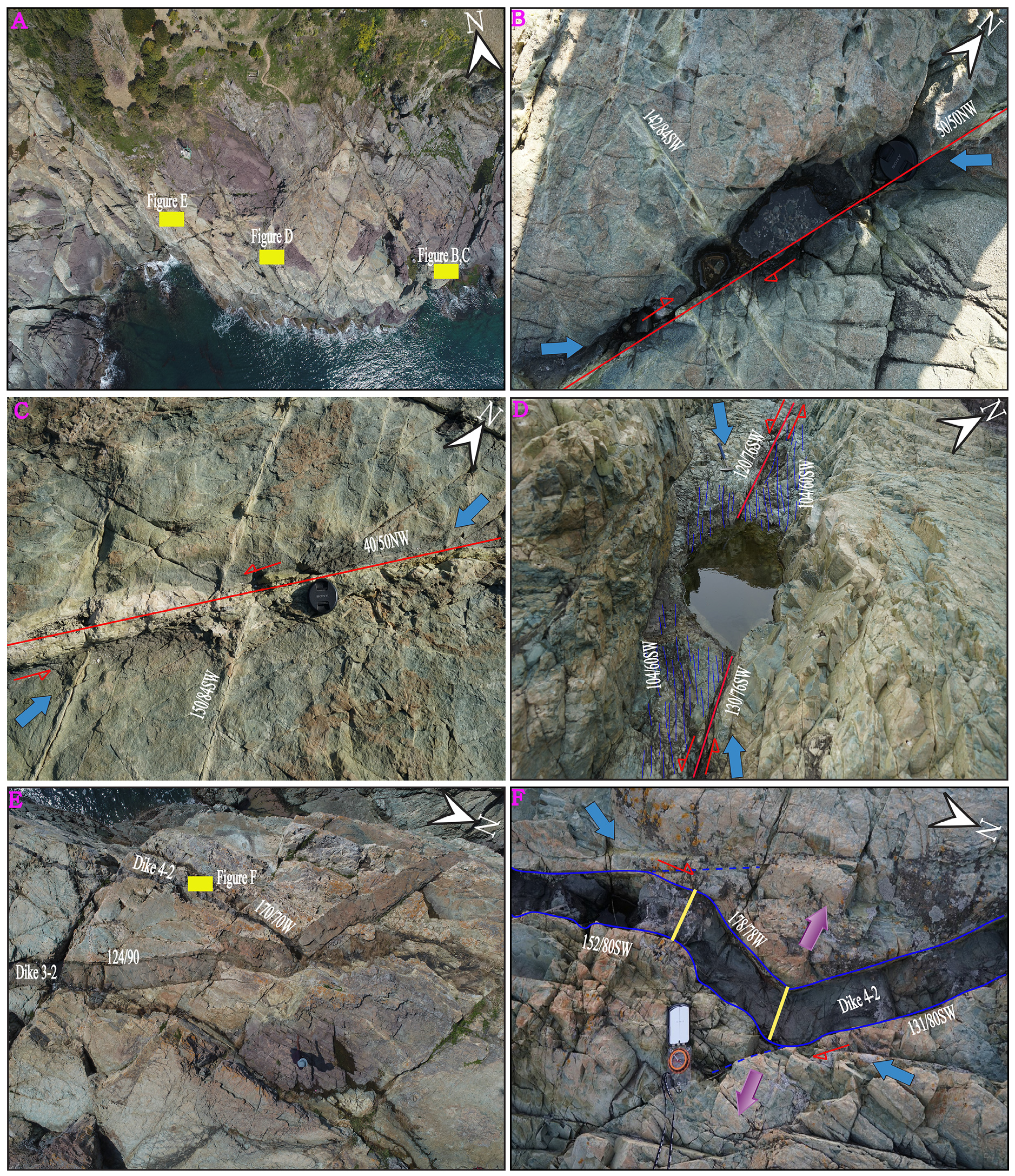
Fig. 4.
Field photographs taken at site 2. A. Field photograph of site 2. B. NE-SW-striking dextral strike-slip fault displacing NW-SE-trending veins. C. NE-SW-striking sinistral strike-slip fault displacing NW-SE-oriented veins. D. Linking zone of fault segments showing a NW-SE-striking sinistral strike-slip fault. E. Dike 4-2 intruding dike 3-2. F. Dike 4-2 intruding along a dextral strike-slip fault. Yellow lines on Fig. 4F indicate piercing points showing the opening direction of the dike. Red and blue arrows indicate the sense of movement and maximum principal stress, respectively. Thick, solid blue lines depict dike contact planes, and red lines represent faults. Thin blue lines show secondary fractures, and dashed blue lines near the piercing points indicate the tips of segment faults.
Site 3
Lithologically, this site is composed of reddish siltstones and shales which were intruded by magmatic dikes (Fig. 5A). Site 3 also shows deformation events, including cross-cutting dikes and faults within dikes. NNE-SSW-oriented dike 1-3 is displaced by a WNW-ESE dextral strike-slip fault with 1 m of displacement (Fig. 5B), and it is intruded by NW-SE-oriented dike 3-3, indicating two separate intrusion events (Fig. 5C). Dike 3-3 is oriented in the same direction as mode I fractures in Figs. 5D and 5E. Mechanically, mode I or opening mode fractures form when the tensile stress is normal to the plane of the crack (Chang et al., 2002). The absence of these fractures in the dike 3-3 indicates that they were formed before the intrusion. Therefore, we suggest that the geometry and orientation of intrusion of dike 3-3 were controlled by pre-existing mode I fractures (Fig. 5F). E-W-oriented fractures near dike 3-3 dip at around 40° and are restricted in location to the dike planes on which there are slip surfaces. Therefore, we suggest that these fractures represent the tip damages of the fault, indicating that faulting occurred after dike intrusion owing to stress perturbation caused by the dike. The model presented in Fig. 5F summarizes three stages of structural development around the dike 3-3: 1) Mode I fractures were formed parallel to the direction of maximum principal stress; 2) dike 3-3 was affected by dilating mode I fractures that formed during stage 1; and 3) stress perturbation caused the formation of a sinistral strike-slip fault around the dike, forming the linking zone of two fault segments (Fig. 5F).
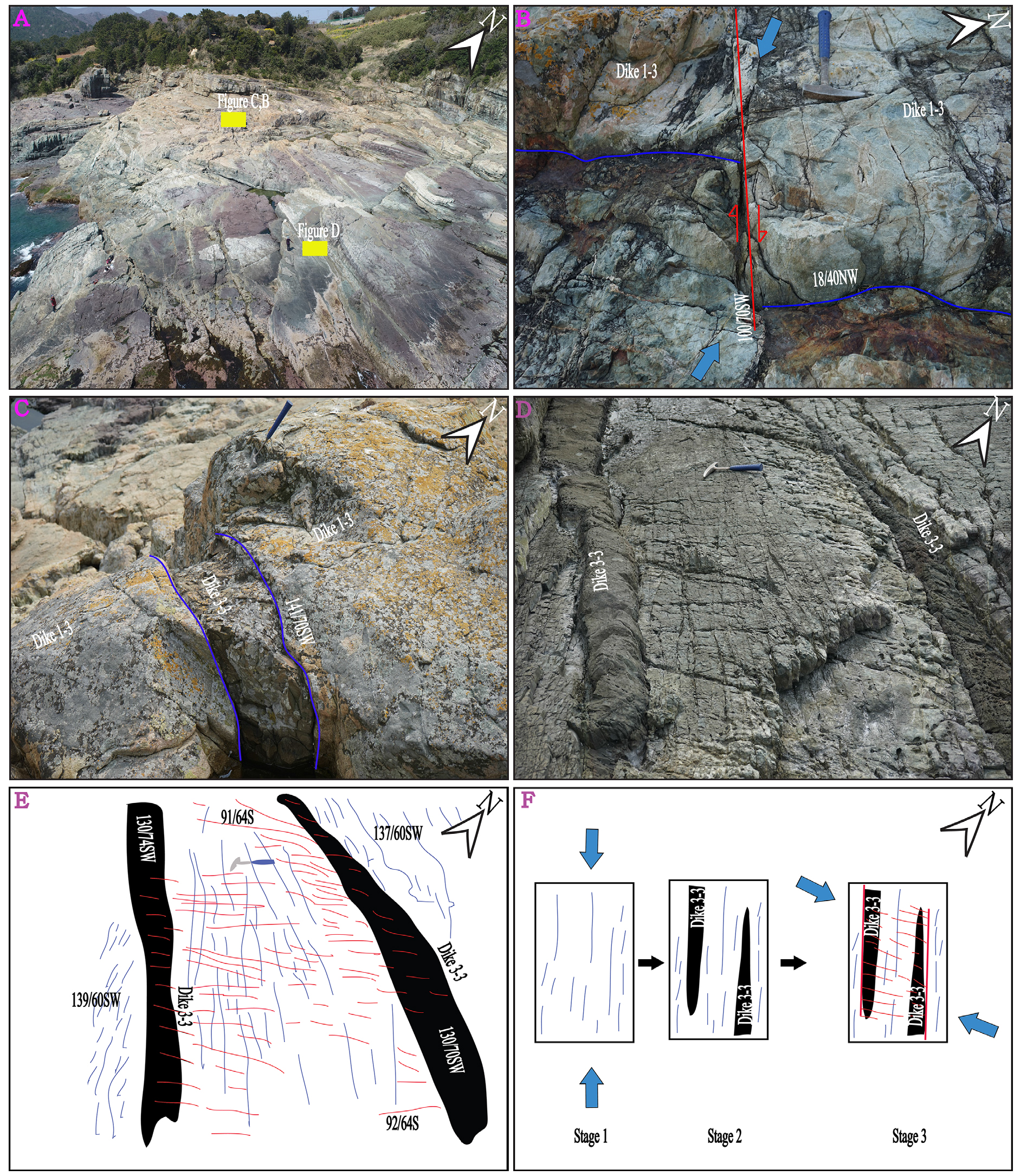
Fig. 5.
Site 3. A. Field photograph of site 3 outcrops. B. Strike-slip fault within dike 1-3. C. Dike 3-3 intruding dike 1-3. D. Dike 3-3 intruding along pre-existing mode I fractures. The dike was later reactivated as a fault. E. Sketch of dike 3-3. F. Model of dike 3-3 emplacement and reactivation. Blue arrows indicate the orientation of maximum principal stress, and red arrows indicate the sense of movement. Thick, solid blue lines indicate dike contact planes, and thick, solid red lines indicate faults. Thin blue and red lines indicate fractures of different orientations.
Discussion
Deformation events restored on the basis of structural data obtained from the study area can be classified into five events, as summarized in Fig. 9. These events were reconstructed on the basis of the orientation of the maximum principal compressive stress analyzed from data on fault slip senses, dike orientations, and opening directions of intruded pre-existing structures. It is commonly accepted that the propagation direction of a dike is parallel to the maximum principal stress and perpendicular to the minimum principal stress (Anderson, 1951). However, if the dike intrudes along pre-existing fractures, the opening direction in some cases does not follow this rule and should be inferred from piercing points on both sides of the dike (Delaney et al., 1986). The piercing points for dike 4-2 are oblique to the strike of the dike rather than perpendicular. Because matching of piercing points provides a more reliable opening direction compared with the perpendicular relationship between dike strike and minimum principal stress, if the opening direction is not perpendicular to the dike strike, it can be assumed that the dike intruded along a pre-existing feature (Delaney et al., 1986; Le Gall et al., 2005). The line connecting the piercing points for dike 4-2 is oblique to the strike of the dike, and the unconnected end parts of the two fault segments indicate a linking damage zone formed by the interaction of those segments. The linking damage zones formed in Figs. 3D, 4D, and 5E can be explained as a result from non-linear basement fault geometries (e.g., Aydin and Nur, 1982; An and Sammis, 1996; Dooley and Schreurs, 2012). Therefore, considering the direction of maximum principal stresses inferred from this dike, we suggest that dike 4-2 intruded along a dextral strike-slip fault (Fig. 4F).
Dikes observed in the study area can be grouped into four main sets based on their cross-cutting relationships and strike directions. These sets are defined by orientation as NNE-SSW, NE-SW, NW-SE, and NNW-SSE from oldest to youngest (Fig. 6). According to relationships between different dikes, NE-SW-oriented dikes are intruded by both NW-SE- and NNW-SSE-oriented dikes. Therefore, the NE-SW-trending dikes are older than the two other sets (Fig. 3A). Furthermore, NW-SE-oriented dikes are intruded by NNW-SSE-oriented dikes, meaning that the former are older than the latter (Fig. 4E).
Faults observed and measured in the field can be classified into four sets based on their strikes: NW-SE, NNW-SSE, NE-SW, and E-W (Fig. 7). The faults are categorized into sinistral and dextral strike-slip faults based on their slip senses. Sinistral strike-slip faults are dominant in the NW-SE, NNW-SSE, and NE-SW sets, whereas dextral strike-slip faults are dominant in the E-W, NW-SE, and NE-SW sets (Fig. 8).
NW-SE-striking sinistral strike-slip faults and E-W-striking dextral strike-slip faults show Andersonian conjugate sets. Conjugates faults cutting dike 2-1 have an intersection angle of 52°, and the structural deformation event occurred after dike intrusion (Fig. 3B). Freund (1974) argued that it is impossible for intersecting conjugate faults to be displaced synchronously. However, Nicol et al. (1995) suggested the possibility of synchronous movement on conjugate faults. Odonne and Massonnat (1992a) argued that offset sets of faults can be caused by a difference in fault length or a difference in the orientation of the fault sets to the major principal strain axis. In other words, a larger slip on one conjugate pair can induce slip on the other pair. In addition, the formation of conjugate faults at low angles of ≤60° may be caused by shear deformation along a pre-existing mode I fracture (Hancock, 1985). Conjugate faults on dike 2-1 show an offset relationship and an intersection angle of ≤60°. Therefore, we suggest that these conjugate faults resulted from shearing along mode I fractures and that the offset along the NW-SE-striking sinistral strike-slip fault was induced by a dextral strike-slip fault.
Results of the present study agree with the findings of the previous investigation by Yang et al. (2008) (Fig. 9) in terms of deformation history of Geoje Island. Yang et al. (2008) similarly identified five structural deformation events in Geoje with respect to the direction of maximum principal stress (NNE-SSW, NE-SW, NW-SE, NNW-SSE, and ENE-WSW) by analyzing dike intrusion patterns.
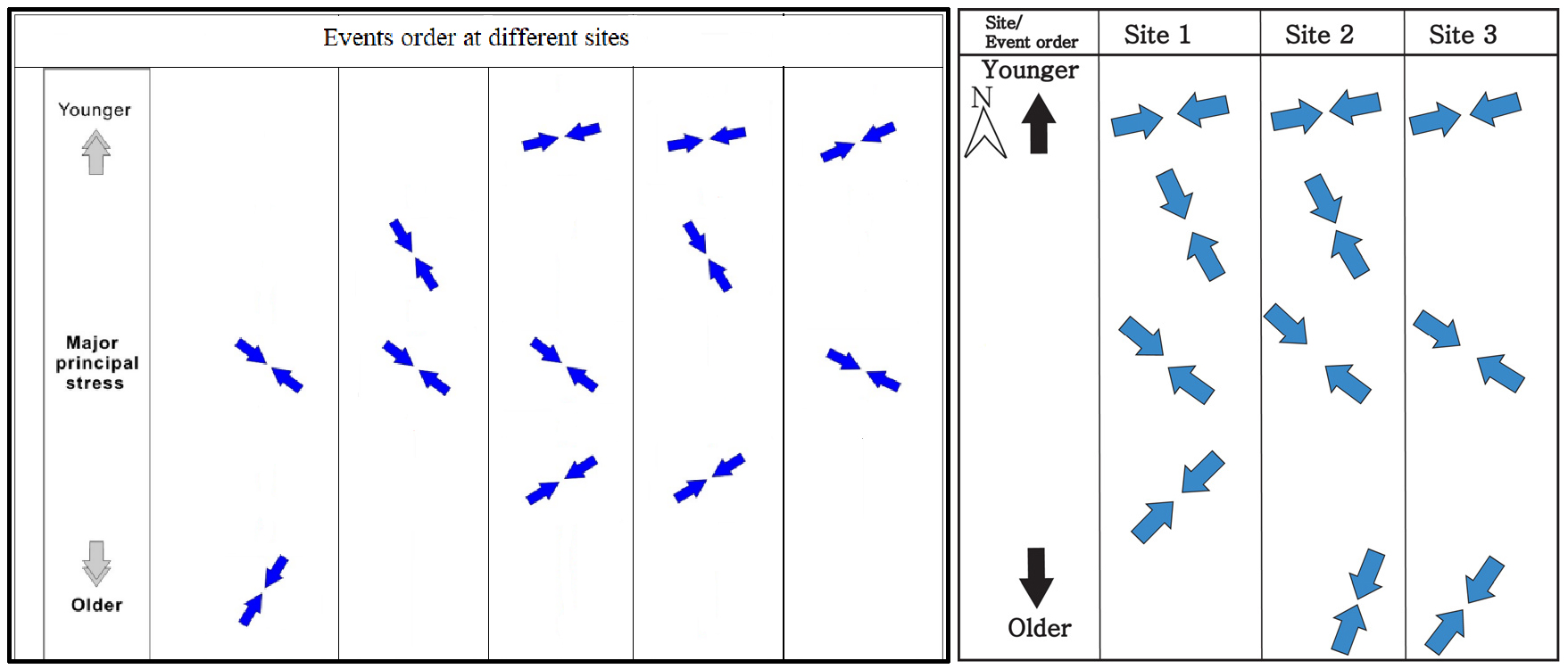
Fig. 9.
Comparison of the deformation history determined in this study (right) with that determined by Yang et al. (2008; left) in relation to the orientation of maximum principal stress. Blue arrows indicate the orientation of maximum principal stress. Gray and black arrows indicate the order of events.
Our study aimed at understanding brittle deformation within sedimentary rock in Geoje Island by combining dike emplacement kinematics and faulting events, and also at deducing the relationship with the tectonic history around Korean Peninsula. In tectonic terms, the brittle deformation events identified in Geoje Island can be related to the reactivation of the NNE-trending Yangsan strike-slip fault system, which is located in the northeastern part of the study area. This proposal is supported by the close relationship between the orientations of maximum principal stresses associated with the reactivation history of the Yangsan fault system, as found in previous studies (e.g. Cheon et al., 2019) and those identified in the present study (Fig. 10), as follows. During the Late Cretaceous, the movement on the YFS was sinistral under NW-SE-directed compression (Cheon et al., 2019). During the Miocene, the movement sense was also sinistral under NW-SE-directed compression. The last event during the Quaternary, the movement on the YFS changed from sinistral to dextral system under E-W- or ENE-WSW-oriented compression, which is the current stress regime on the Korean Peninsula (Jun, 1991). The remaining first two events of NNE-SSW- and NE-SW-directed compression in this study may have occurred before the events presented in Fig. 10. After the Late Cretaceous, the direction of subduction of the Pacific plate beneath the Eurasian plate changed from NNW to NW (Fig. 10). We suggest that this change in the direction of subduction of Pacific plate induced stresses on Korean peninsula, hence the reactivation of Yangsan Fault System affected the deformation of sedimentary rocks in Geoje Island.
Given the above, we conclude that the brittle deformation of rocks in Geoje Island was caused by episodic reactivation of the Yangsan fault system. The reactivations of the fault system were likely a result of changing tectonic interactions among the Philippine Sea, Pacific, and Eurasian plates, and/or changes in the angle of subduction of the Pacific plate beneath the Eurasian plate (Cheon et al., 2019). More detailed evidences would be needed to confirm the relationship between Yangsan fault system reactivation and these plate interactions and processes.
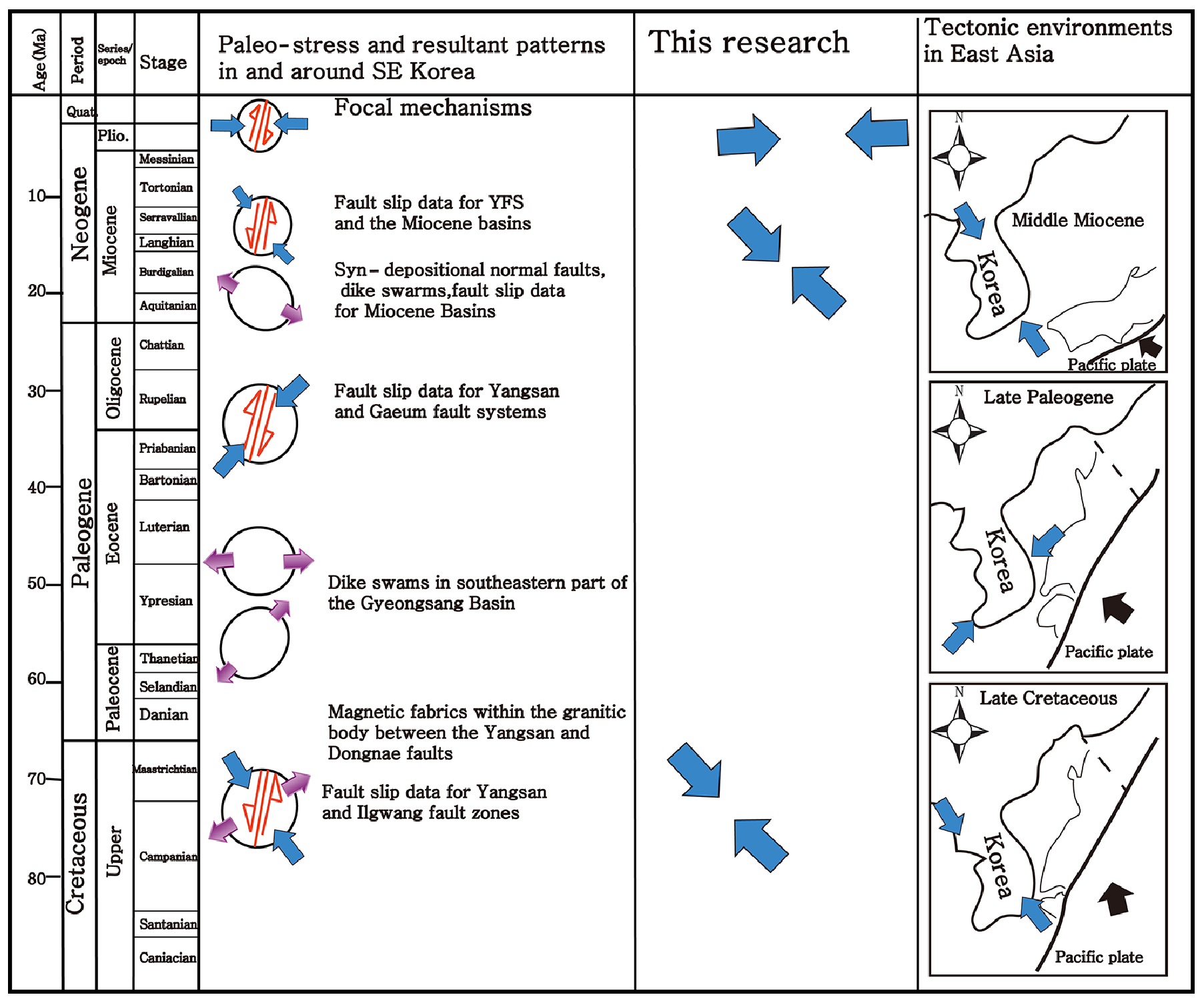
Fig. 10.
Proposed relationship between structural deformation events on Geoje Island and movement on the Yangsan fault system, as well as the relationship with the regional tectonic setting on and around the Korean Peninsula (modified from Cheon et al., 2019). Blue arrows indicate the direction of maximum compressive stress, purple arrows indicate the orientation of minimum principal stress, black arrows show the direction of plate subduction, and red arrows indicate the direction of movement on the Yangsan fault system. Red lines depict the Yangsan fault system.
Conclusion
This research identified structural deformation events from kinematic analysis of dikes and structures developed within sedimentary rocks in Geoje Island, southeast Korea. Dikes commonly intrude in the direction perpendicular to the minimum principal stress, but this rule changes when dike intrusion is controlled by pre-existing structures where the opening direction is oblique to the main strike direction of the dike. Our study shows that the geometry and orientation of some dike intrusions in Geoje Island were controlled by pre-existing structures (e.g., faults and mode I fractures), whereas others created their own intrusion pathways. The brittle deformation results obtained from Geoje Island allowed five deformation events to be determined on the basis of the direction of the maximum principal stresses and cross-cutting relationships. These events involved maximum principal stresses oriented NNE-SSW, NE-SW, NW-SE, NNW-SSE, and E-W from early to late. The structural deformation events in the study area were closely related to episodic reactivation of the Yangsan strike-slip fault system (located in the northeastern part of the study area), as established by the high degree of similarity between the known deformation/movement history of the Yangsan fault system and the structural data and inferred deformation events of the present study. In a wider tectonic context, temporal changes in the angle and direction of Pacific plate subduction under Eurasia might mainly affect the changes in the stress field of Geoje Island, which led to the episodic reactivation of the Yangsan fault system and the different deformation events in the island. Regional tectonic controls on the deformation of Geoje Island should be more thoroughly investigated in future studies.