Introduction
Geological Setting
Results and Discussion
Rainfall Characteristics
Landslide Characteristics
Mineralogical Characteristics of Soils
Possible Failure Mechanisms
Conclusions
Introduction
Due to extreme rainfall for three consecutive days, at UTC+9 20:30 on 7 August 2020, a catastrophic landslide occurred at the Osan village, Gokseong County, South Jeolla Province, South Korea (Fig. 1a and 1b). A reservoir lies 478 m away from the landslide crown (Fig. 1a), and an irrigation stream is adjacent to the landslide toe (Fig. 1c). The location of the landslide scar (35°11'42.29"N, 127° 8'10.21"E) was on a part of the National Road No.15 (Fig. 2a), which was supported by a retaining wall (Fig. 2b and 2c). The Gokseong landslide was triggered or primed by intense rainfall, and then transformed into a debris flow. The slip surface of the slope failure included part of the traffic road lane, retaining wall and road embankment slope, which were under road-widening work (Choi et al., 2021). Despite the relatively low elevation and gentle slope, the high-speed and long-runout landslide damaged 5 houses and buried 5 residents. The sliding direction was approximately 343°. In the travel path of the debris flow, the gully is in V-shape in the upper part near the source area and in U-shape from the area of a damaged car (Fig. 2d). The erosion, about 1.2 m in depth and 0.3~0.4 m in width, was found in the middle and lower part of the debris flow gully (Fig. 2e). Several retaining walls and residential buildings were damaged by the debris flow (Fig. 2f and 2g).
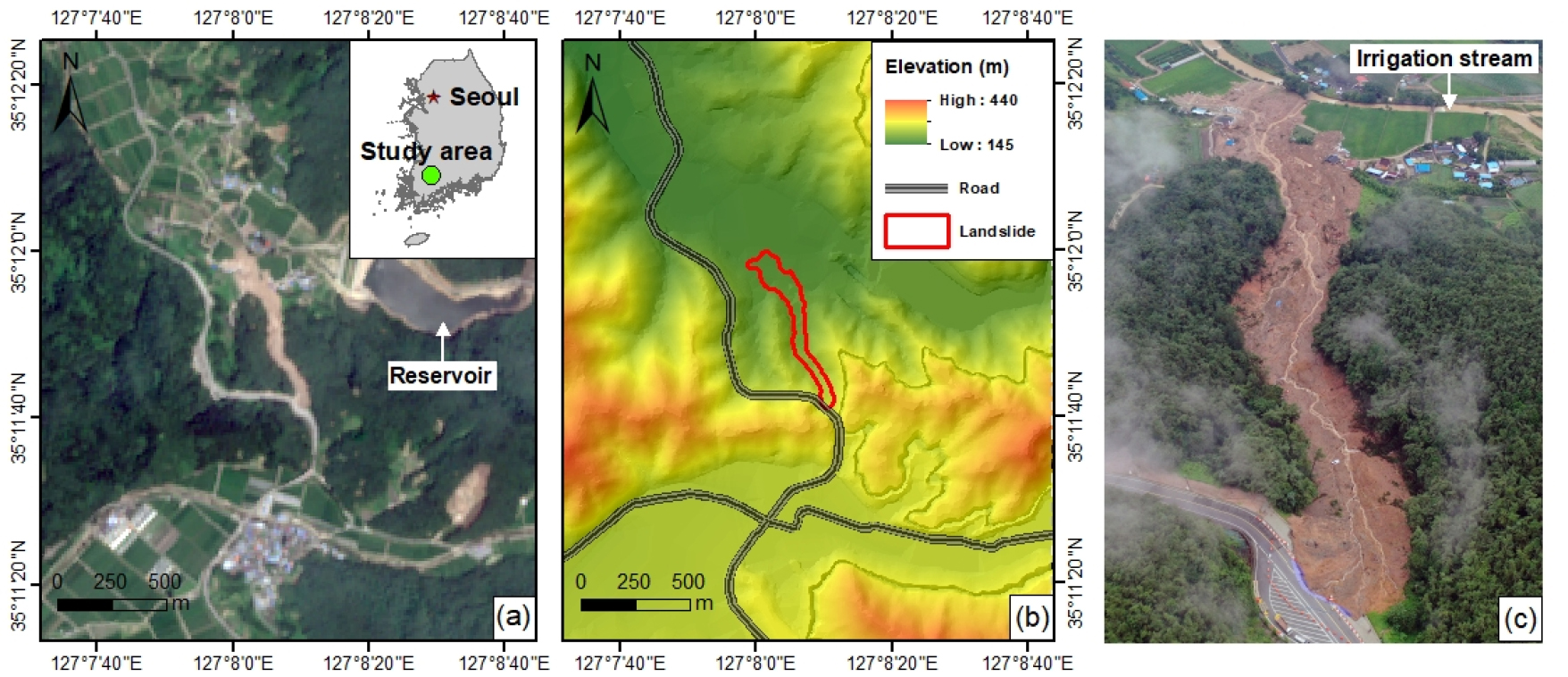
Fig. 1.
Location map of the Gokseong landslide occurred on 7 August 2020. (a) RGB color composite (band 4/band 3/band 2) of Sentinel 2 satellite image acquired on 20 August 2020 (https://scihub.copernicus.eu/), (b) Elevation, road network, and landslide zone, (c) a photo taken by Korea JoongAng Daily showing a collapsed road and debris flow.
Geological Setting
The crown of the Gokseong landslide was located on the mountain ridge, which was passed by the National Road No. 15. The upper area of the landslide is dominated by conglomerate, sandstone, and tuff covering the granite bedrock. The valley is a folded structure in the N30W direction (Son and Kim, 1966). The landslide moved along the valley of the syncline structure in the direction of N17W between the andesitic lava flow erupted in the Cretaceous Period of the Mesozoic era and granite of unknown age (Fig. 3). The landslide was deposited in the boundary of the irrigation stream channel in the Quaternary alluvial fan. From a geological perspective, the main flat bottom valleys in Gokseong County are composed of deeply weathered rocks (Choi et al., 2021). The characteristic weathering profiles of these granitic and dioritic rocks are grus and laminated grus with the thicknesses of several tens of meters. Below the loose weathered materials, fissured zones with irregular orientations are present. Iron-stain coatings have also formed due to water percolation. Inferred grabens with well-preserved weathering covers are present on site (Choi et al., 2021).
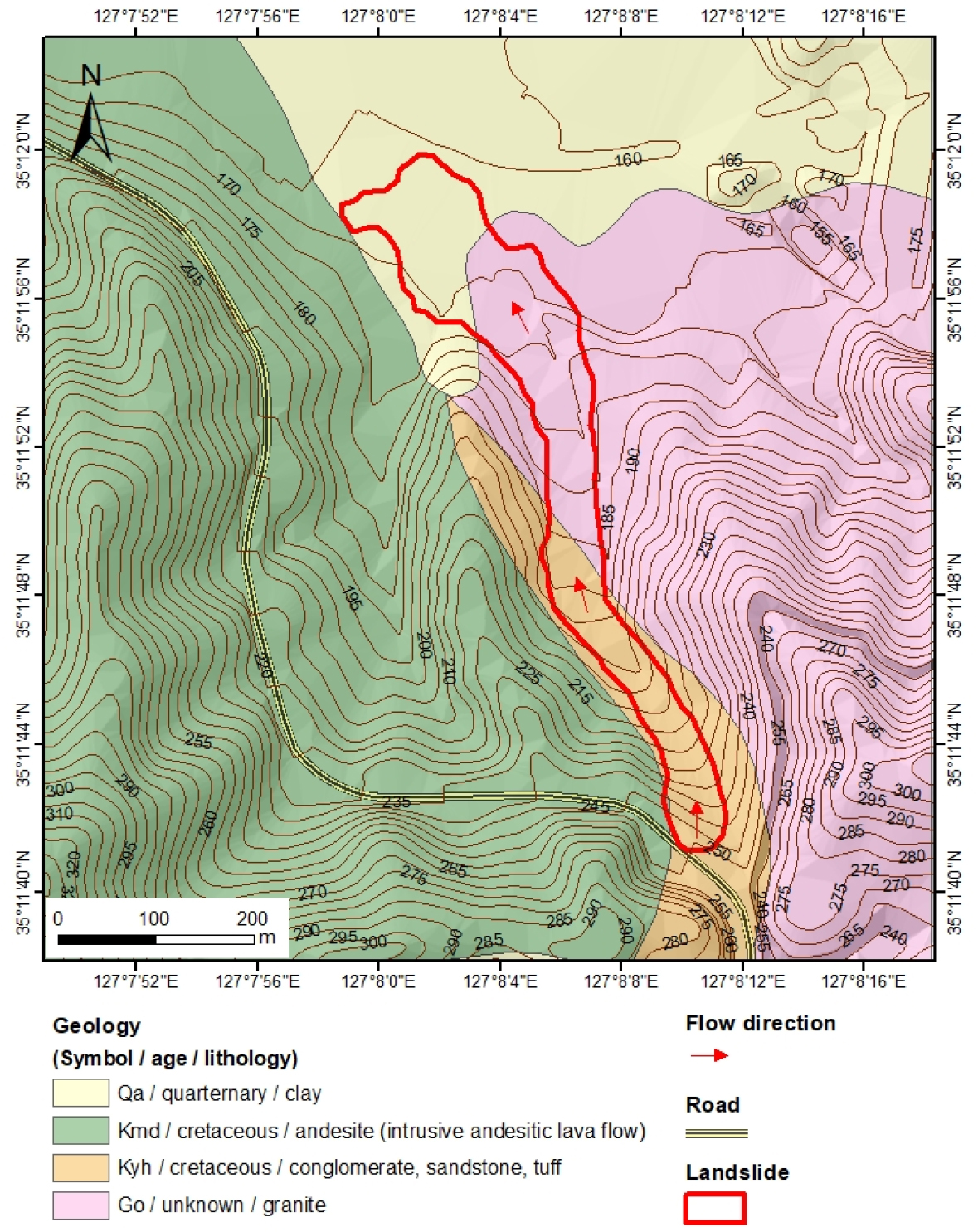
Fig. 3.
Geological map of the landslide. The geology condition is determined based on a 1:50,000 scale geological map by Korea Institute of Geoscience and Mineral Resources (https://data.kigam.re.kr/). Pre-landslide elevation was obtained based on 1:5,000 scale map by Korean National Geographic Information Institute (https://www.ngii.go.kr/). The main body of debris flow is parallel to the NNW-SSE direction of Kyh with conglomerate, sandstone, and tuff covering granite bedrock.
Results and Discussion
Rainfall Characteristics
In South Korea, it has long been known that precipitation is not evenly distributed throughout the season, and the rainy and dry seasons are well-defined. Most of the annual rainfall is concentrated in the summer ranging from June to September, especially at the beginning of August when the tropical storms frequently occur. Climatologically, the southern part of the Korean peninsula experiences the Asian monsoon climate (Jung et al., 2020). Nearly half of the annual precipitation of 1,350 mm falls with summer typhoons and rainstorms (Jung et al., 2020). Fig. 4 illustrates the isohyet map of the cumulative rainfall for 72 h during the period of 5 to 7 August 2020. It shows that the Gokseong landslide occurred in the area with intense rainfall, where the cumulative rainfall was greater than 270 mm. The cumulative rainfall was recorded by the AWS 755 meteorological station located 7 km away from the landslide area, with an amount of 274 mm (AWS; Automatic Weather Station, https://data.kma.go.kr/). The highest hourly rainfall was 52 mm at the beginning of August 2020 (Fig. 5).
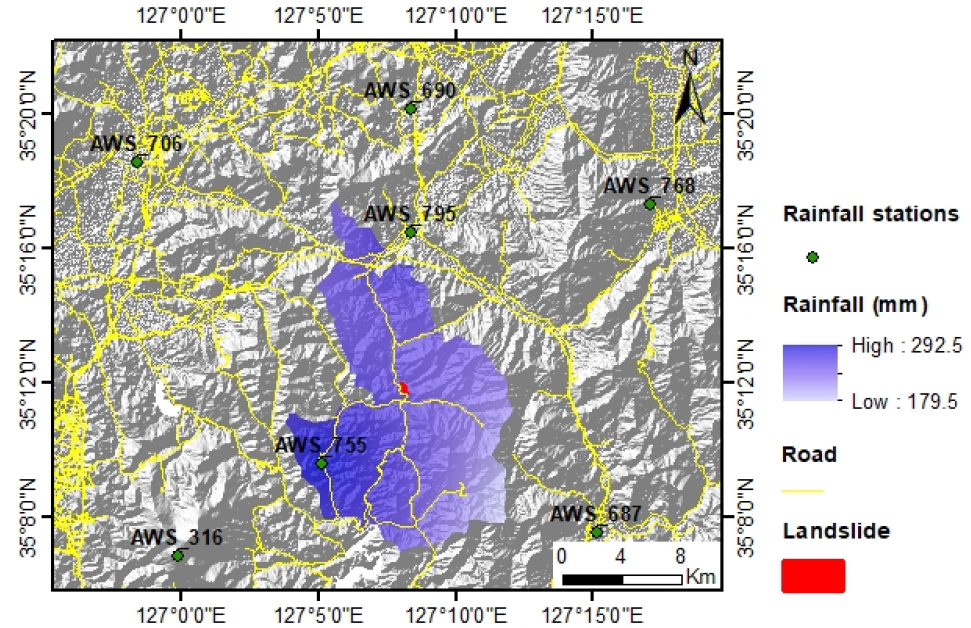
Fig. 4.
Isohyetal map of cumulative rainfall from 5 to 7 August 2020 using 7 rainfall stations from AWS (Automatic Weather Station) of Korea Meteorological Administration (https://data.kma.go.kr/). Yellow lines describe road network around the landslide.
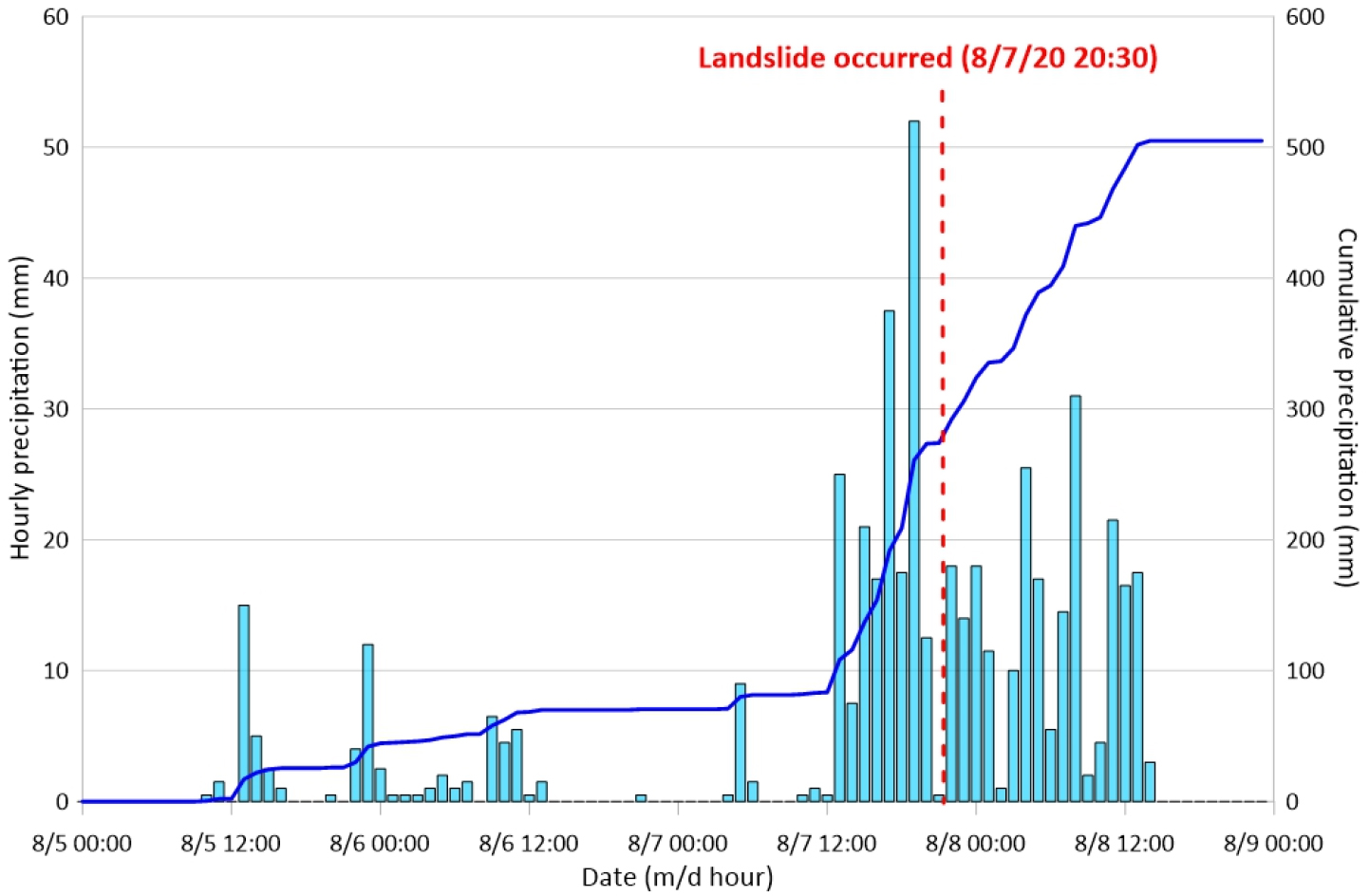
Fig. 5.
Hourly rainfall recorded from 5 to 9 August 2020 from the AWS 755 station located 7 km away from the landslide site. On 7 August 2020, the cumulative rainfall was 274 mm at 21:00 and the maximum hourly rainfall was 52 mm at 19:00 (https://data.kma.go.kr/).
Landslide Characteristics
Fig. 6 illustrates the longitudinal profiles that were measured at nearly 10 m intervals by Nikon Laser Rangefinder 550AS and compared with the DEM (Fig. 6a) obtained before the landslide by the Korean National Geographic Information Institute (https://www.ngii.go.kr/). Based on the geological and geomorphological conditions, the landslide can be divided into three zones: initial sliding zone I, energy dissipation zone II, and deposition zone III. The main body of the landslide has a total length of 640 m and a traverse width of about 50~120 m. The landslide crown has a slope angle of 45.6°, a width of 62 m, and a failure depth of 12 m. The source area of the debris flow is composed of clay-rich soils covering granite bedrock, which results in an acceleration in the initial sliding zone (Fig. 6b). The weathered soil layer is composed of conglomerate, soil, and silt with thickness of 8~12 m. In the exposed granite bedrock, sheeting joints and micro-sheeting joints are well developed along the NNW-SSE direction parallel to the slide. The area where a damaged car is placed was estimated to be the energy dissipation zone (Fig. 6c). In the middle of the debris flow path, the gully bed was composed of loose and unstratified soil matrix, with fragments from the retaining wall and road. The weathered soil layer is thin, with thickness of 0.7~1.5 m. The toe is located near the irrigation stream channel, which is structurally controlled in the deposition zone (Fig. 6d). The gully erosion and spring water were found at the beginning of the deposition zone. Debris flow was deposited from a damaged house to the irrigation stream channel.
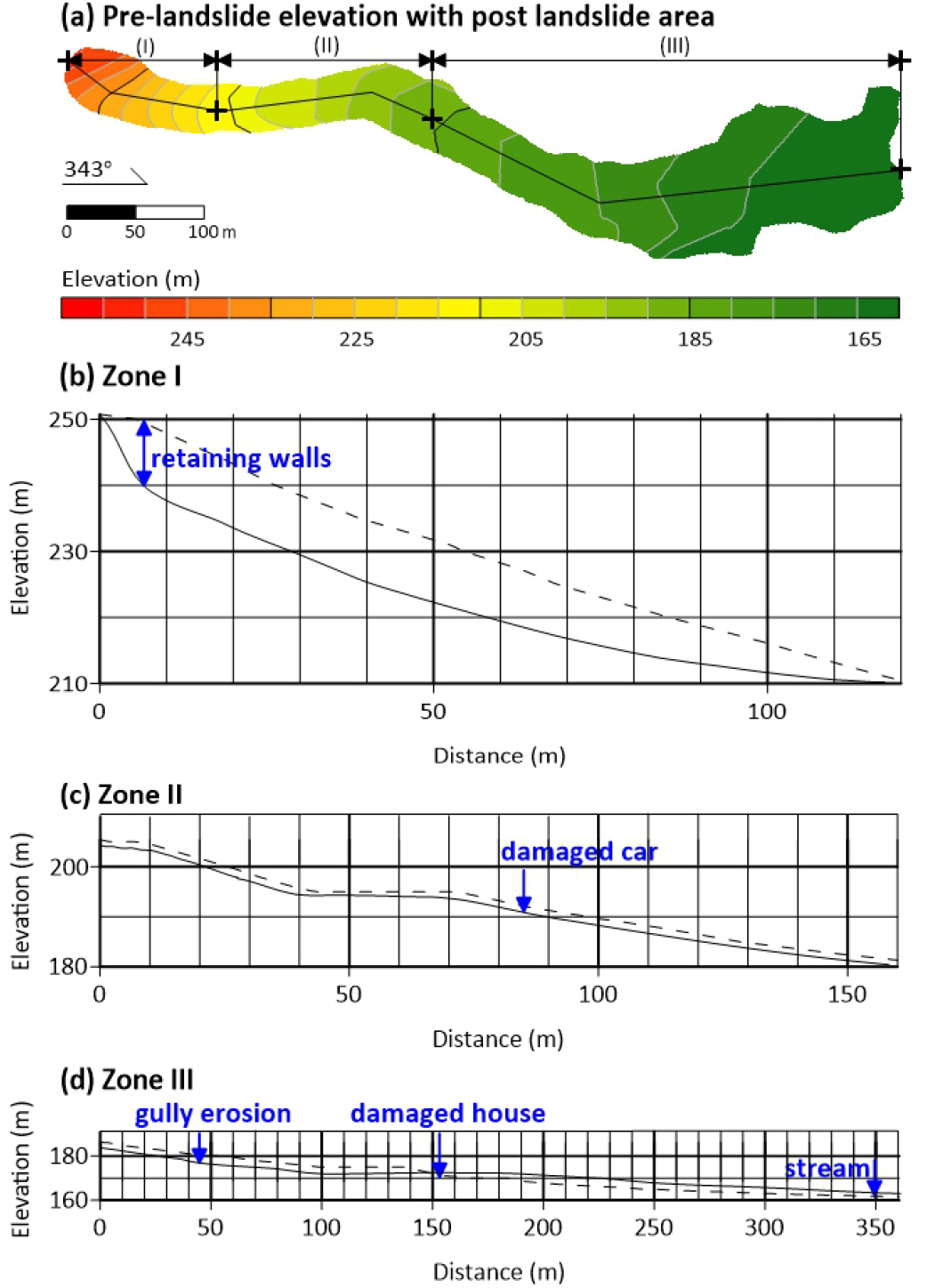
Fig. 6.
Longitudinal sections of the Gokseong landslide. (a) Pre-landslide elevation with post landslide area, (b) Zone I: initial sliding zone, (c) Zone II: energy dissipation zone from a damaged car, (d) Zone III: deposition zone from damaged house. The dotted line is from 1:5,000 scale topographic map by Korean National Geographic Information Institute (https://www.ngii.go.kr/) and the solid line is based on the field investigation by Nikon Laser Rangefinder 550AS.
Mineralogical Characteristics of Soils
Soil samples covered on granite bedrock were obtained for laboratory testing. X-ray diffraction (XRD) analysis was conducted on the soil samples (Fig. 7). The analytical conditions of XRD were at a range of 5~65° 2θ at 40 kv/30 mA using XRD (Ultima IV, Rigaku) equipped with a Cu target and Ni filter at the Central Laboratory of Andong National University. After obtaining reflection data from powdered samples, quantitative analysis was conducted on constituent minerals in soil samples using Siroquant v.3.0 (Sietronics in conjunction with CSIRO), as a quantitative analysis program based on Rietveld method using diffraction patterns. For chemical analysis on bulk soil samples, X-ray Fluorescence Spectrometer (XRF ZSX PrimusⅡmodel) was used. After obtaining the chemical data, chemical indices such as Eq. (1) chemical index of alteration (CIA) and Eq. (2) chemical index of weathering (CIW) were calculated (Wang et al., 2015; Baldermanna et al., 2021).
Microtextural observation and semi-quantitative chemical analysis were performed on untreated fresh portions of the soil samples using scanning electron microscopy (SEM, VEGA II LMU model) equipped with energy dispersive spectroscopy (EDS). The analytical conditions for SEM observation were 20 kV and 10 nA using bulk samples coated with Osmium (Os) to avoid peak overlap among specific elemental spectra for the EDS analysis. Although vermiculite and kaolinite, together with quartz and feldspars, are common in the investigated soil samples, mineral constituents in soil samples are similar but variable in their quantity depending on samples (Table 1, Fig. 8). For example, G1 contained quartz 32.1%, albite 20.1%, microcline 26.3%, vermiculite 6.4%, and kaolinite 5.0%, and G2 contained quartz 19.8%, albite 24.7%, microcline 31.4%, vermiculite 7.4%, and kaolinite 16.7%, while G3 contained quartz 30.7%, albite 5.9%, microcline 25.3%, vermiculite 5.9%, and kaolinite 14.5%.
Table 1.
Mineral constituents in soil samples based on XRD quantitative analysis (unit: wt%)
Species | G1 | G2 | G3 |
Quartz | 32.1 | 19.8 | 30.7 |
Vermiculite | 6.4 | 7.4 | 5.9 |
Kaolinite | 15.0 | 16.7 | 14.5 |
Albite | 20.1 | 24.7 | 23.6 |
Microcline | 26.3 | 31.4 | 25.3 |
Chemical weathering indices such as chemical index of alteration and chemical index of weathering were calculated using bulk chemistry (Table 2). CIA and CIW were 74.1, 84.6 in G1 sample, 74.0, 84.5 in G2 sample, and 75.0, 84.7 in G3 sample, respectively. In summary, chemical indices were slightly low in G2 sample, and little difference were found among the soil samples. In addition, iron and alkaline elements are slightly high. Microtextural characterization of bulk soil samples was investigated using scanning electron microscope (SEM) equipped with energy dispersive spectrum (EDS). For instance, G1 sample showed that grains were loosely interlocked or arranged randomly (Fig. 8). Main constituent minerals in the soil are quartz, vermiculite, illite, and smectite. Quartz surface are partly corroded, and clay minerals such as vermiculite and illite are characterized by flaky grains stacked. Soil structures vary in size and shape though they are composed of nearly similar mineralogy. In G2 sample, clay minerals and fine-grained crystals were intimately arranged (Fig. 9). Although there are some trace minerals identified by XRD, main constituent minerals are illite and smectite, Clay minerals are characterized by flaky or thinly crystals. In G3 sample, most grains in soil samples are arranged with primary minerals such as quartz and feldspars (Fig. 10). Vermiculite is the most common clay mineral that surrounds quartz grains, or the former is closely associated with the latter. K-feldspar showed alteration texture and quartz exhibited weak corrosion texture.
Table 2.
Bulk chemistry of soil samples analyzed by XRF analysis (unit: wt%)
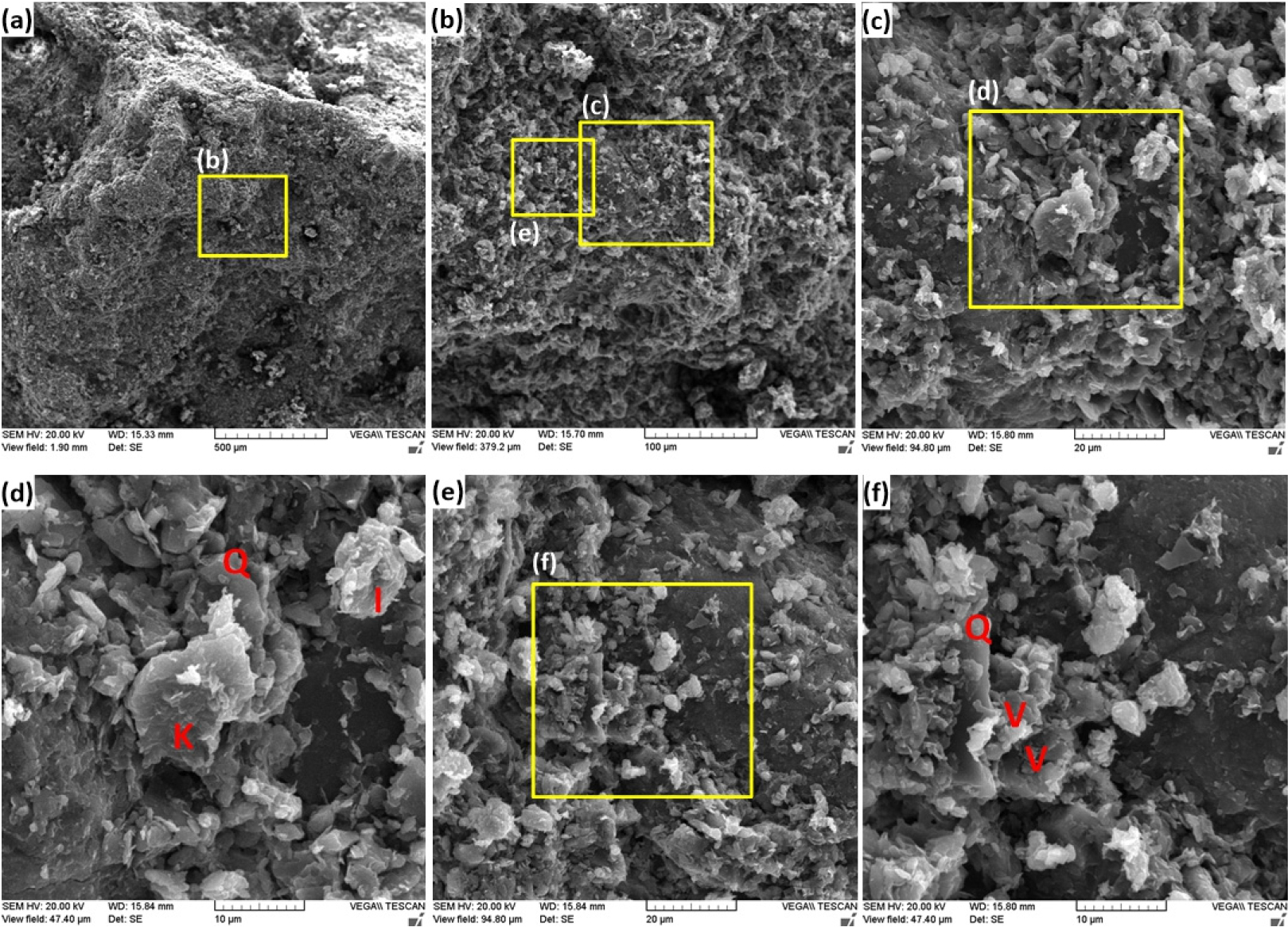
Fig. 10.
SEM images of G3 soil sample. (a) low magnification (×100), (b) enlarged image of the box area in (a) (×500), (c) enlarged image of the box area in (b) (×2,000), (d) enlarged image of the box area in (c) (×4,000). (e) clay textures (×2,000), (f) enlarged image of the box area in (e) (×4,000). I: illite, K: kaolinite, Q: quartz, V: vermiculite.
Possible Failure Mechanisms
The main triggering factor of the landslide is extreme rainfall for three consecutive days. In addition, the possible failure mechanisms based on the detailed field investigation and chemical analysis can be summarized into five different points.
(1) Road construction: Before the landslide occurred, blasting on slopes was carried out for the expansion of National Road No.15, and its pile of soil was deposited in the landslide crown (Fig. 11). As a result, it is estimated that the overload of the soil dummy and the lack of drainage system affected the Gokseong slope stability.
(2) Seepage: The landslide zone is a catchment area that accumulates water flow below the mountain ridge. Seepage was clearly observed not only in the source area but also in the whole landslide body (Fig. 11a and 11b). Most of the long-runout landslides have high potential energy and steep slopes in the source, which results in a rapid landslide. Despite gentle slope after acceleration zone of 120 m (Fig. 11c), ongoing seepage from the source area of steep slope might play an essential role in controlling a long-runout landslide in this study area.
(3) Geological structure: The fold typically forms during crustal deformation as the result of compression that accompanies mountain building. This study area is a syncline structure corresponding to the valley of the folded strata. The landslide flowed along the valley on both sides between two different geological layers and the direction of joints of strongly weathered granite bedrock (Fig. 11d). From the geological perspective, the geological structure is one of the main factors affecting a high-speed and long-runout landslide.
(4) Swelling pressure: Once the moisture content rises, the horizontal swelling pressure as horizontal effective stress will increase, and as wetting fronts move downward, resulting in the increasement of the vertical deformation of swelling clay soils.
According to Aksu et al. (2015), during the infiltration of water at the ground surface, swelling pressure for horizontal effective stress increases immediately as moisture content rises. Progressive infiltration during the rainy season keeps clay soils above the water table in a swelled state, and pore water pressures along the shear boundaries of landslide increase, reducing frictional resistance with sufficient infiltration. Besides, landslides on gentle slopes can be caused by cyclic shearing-induced liquefaction of saturated and unconsolidated granular materials (Watkinson and Hall, 2019). The long-runout landslide like the Gokseong landslide was speculated as not only swelling pressure according to the stress relief resulting from the collapse of the road embankment but also excess pore water pressure weakening the soil strength due to ongoing infiltration.
(5) Chemical weathering: Swelling clay soils, including illite and smectite, have serious effects on slope stability (Carrière et al., 2018). This study area is highly sensitive to variations in water chemistry. Seasonality-driven interaction between Na+-loaded water and the pelitic sediments promotes exchanges of Na+ for Ca2+ and Mg2+ ions in the interlayer of smectite, causing a destabilization of the clay matrix (Baldermanna et al., 2021). Vermiculite exhibits a crystalline structure 2:1, and is in the same group of the smectites, including montmorillonite, beidellite, hectorite, saponite, and other varieties. Vermiculite is differentiated from other expandable 2:1 clay minerals by a high negative electrostatic layer charge, due to a higher substitution of Si4+ for Al3+ in tetrahedral sites (Brigatti et al., 2005; Tjong, 2006). Layer charge significantly influences several physicochemical properties of clay minerals, such as cation-retention capacity, in addition to the adsorption of water and various polar organic molecules; swelling or sedimentation volumes; rheology, and others (Christidis et al., 2006; Zemanová et al., 2006; Dultz et al., 2012). Naturally occurring vermiculites exhibit crystalline swelling, but not osmotic swelling. However, despite the high layer charge, vermiculites can exhibit a considerable osmotic swelling, similar to smectites, when saturated with Li+ or with certain organic cations (Braganza et al., 1990). This phenomenon is associated with the increase in the interlayer space of the clay structure and it is commonly observed in the presence of water. Clay swelling occurs when the clay is dispersed in a solvent or when it is in direct contact with an atmosphere having a high vapor pressure of solvent. The formation of dispersive clays reduces the shear strength and induces spontaneous landslides, especially after heavy rainfall events. Besides, the volume and the residence time of the water infiltrating the underground control the intensity and direction of mineral-fluid interface processes in the pelitic sediments, affecting the slope stability and deformation rate of the sliding mass (Nam et al., 2016). As shown in Fig. 11e and 11f, the clay layer covers the bedrock and the boundary between clay soils and granite bedrock is clearly observed. The clay-rich soils are covered on granite bedrock from the rice paddy field of landslide deposition area to landslide crown. In the case of landslides such as the Gokseong landslide in which the content of expandable clay minerals is relatively high, the swelling clay soils may be a key factor. Landsliding thus may be in response to initial failure at the retaining walls, supported by the swelling pressure and seepage in this region.
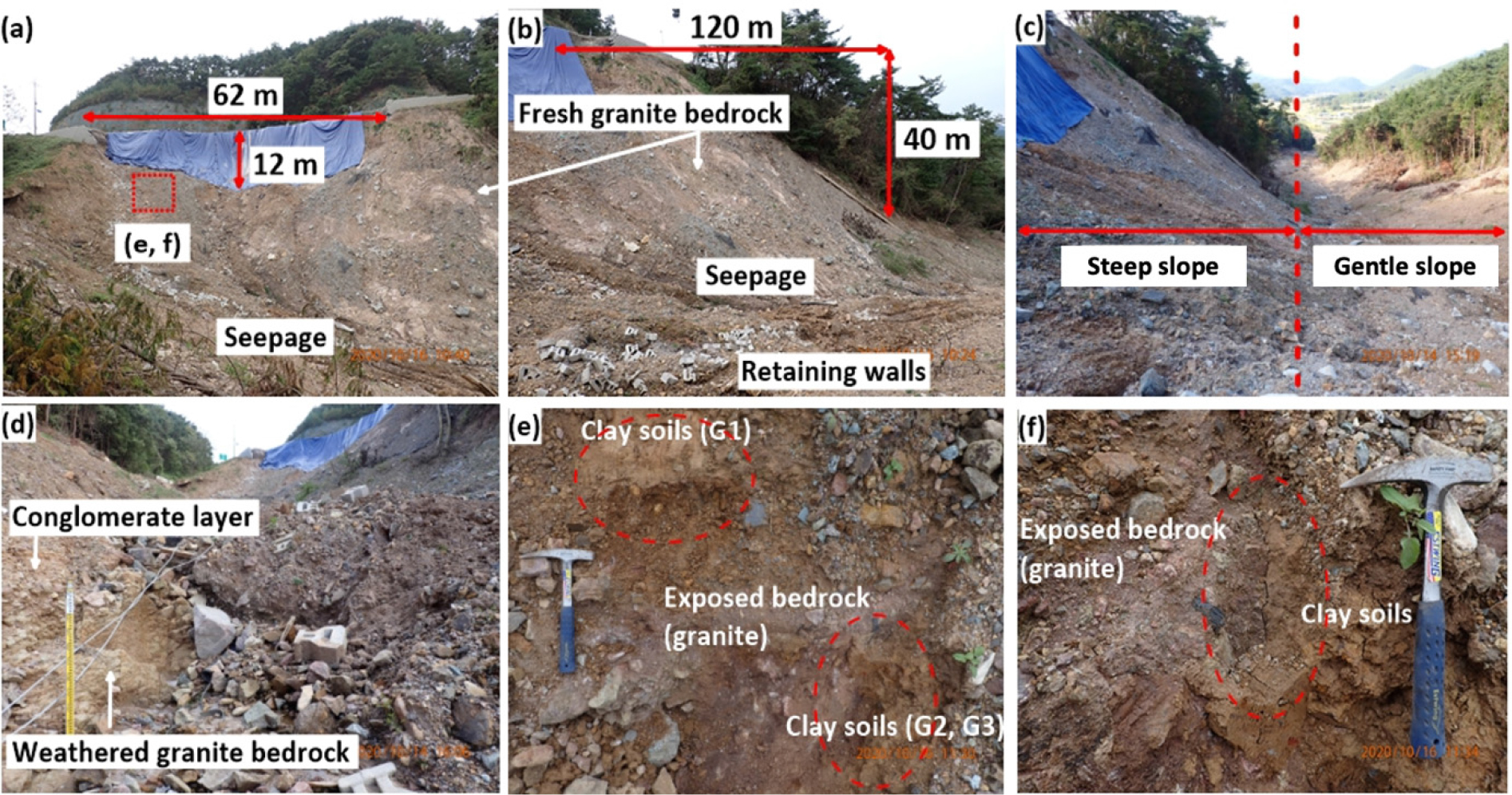
Fig. 11.
Photographs of the Gokseong landslide showing possible failure mechanisms of the long-runout landslide. (a) Width and failure depth of the main scarp, (b) Granite bedrock exposed and seepage at the slope, (c) Pathway of a gentle slope after a steep slope of 120 m, (d) Conglomerate and clay soils covering strongly weathered granite bedrock exposed, (e-f) clay soil sampling at the boundary on clay soils deposited on granite bedrock.
Conclusions
The Gokseong landslide is a rainfall-induced long-runout landslide. Its initiation mechanism is different from the typical landslides reported in South Korea, and the fragments of a retaining wall was transformed into a debris flow. Continuous rainfall with unique geomorphological and geological conditions led to a catastrophic landslide hazard. Through the investigation it was found that Gokseong’s landslide provides further warning that swelling clay soils in mineralogical conditions may critically undermine very gentle slopes, particularly in urban settings. The safety of retaining walls and the construction areas should be sought very seriously during the rainy season in summer. Especially, tropical cyclones with severe storms occurred at the beginning of August in South Korea and, therefore, landslide prevention for the landslide vulnerable zone should be implemented.